This story is contributed by Josh Lehman, Relyion Energy
- Second-life batteries present an immediate opportunity, the viability of which will be proven or disproven in the next few years.
- Second-life batteries can considerably reduce the cost as well as the environmental impact of stationary battery energy storage.
- Major challenges to second-life deployment include streamlining the battery repurposing process and ensuring long-term battery performance.
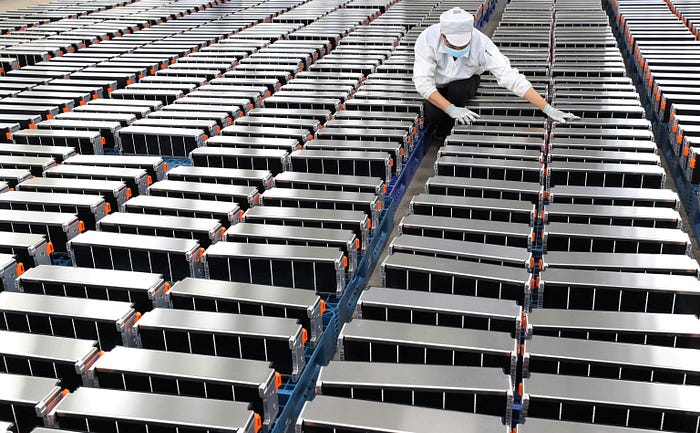
The Opportunity of Second-life Batteries
By 2030, the world could retire 200–300 gigawatt-hours of EV batteries each year. A large fraction of these batteries will have 70% or more of their original energy capacity remaining. This begs the question: does it make more sense to repurpose these batteries for second-life applications, or to throw them in the shredder and extract their raw materials?
The potential to use “second-life” batteries in stationary battery energy storage systems (BESS) is being explored by several startups, along with some grant programs and a few EV manufacturers. On the surface, the value proposition is clear: second-life battery modules could significantly reduce the cost of energy storage, while providing significant environmental benefits by reducing demand for manufacturing and raw material extraction. But challenges exist to cost-effectively repurpose batteries and ensure their performance.
Benefits of Second-life Batteries
Despite the plunge in lithium prices in 2023, first-life batteries still cost 2–6 times as much as second-life batteries. As 2023 drew to a close, first-life LFP modules cost $90–120 per kWh, while retired batteries typically sold for $0–60 per kWh.
But how does this translate into the overall cost of an installed BESS?
Low-cost modules have multiplicative effects, since the module cost savings also reduce inventory costs, warranty and replacement costs, and other lines in a system integrator’s cost structure. On the flip side, depending on the specific degradation, make, and model of battery packs used, a second-life BESS may fit fewer usable kilowatt-hours into the same container. This means a given project may need more containers, which increases balance-of-system costs, construction costs, and siting requirements. (Note that the opposite is also sometimes true: the high energy density of some EV batteries may allow for more energy in a container than traditional ESS modules.)
The cost comparison also depends on project scale: for residential systems, battery modules are a small portion of overall project costs, so the use of second-life batteries is less impactful. The second-life supply chain is rapidly maturing, but today it is unable to serve many utility-scale projects. Therefore, second-life pioneers have largely focused on commercial, industrial, or community-scale applications in the range of 500 kWh up to 20 MWh. At this scale, a fully-installed, 5 MWh second-life BESS will usually cost around $375,000-$750,000 less than traditional, first-life BESS.
Second-life applications also have the potential to improve profits for recyclers. A 2023 report by McKinsey estimated that, by 2025, battery recyclers could generate $600 of value for every ton of batteries processed. A savvy battery recycler, on the other hand, could generate up to $9,000 of value per ton by reselling battery packs with second-life potential.
Second-life batteries carry additional societal benefits. From a carbon perspective, one megawatt-hour of second-life batteries represents approximately 450 metric tons less embodied carbon than a megawatt-hour of first-life batteries.
This carbon intensity comes largely from manufacturing, although the two main methods of lithium extraction have significant environmental footprints as well: brine extraction requires about half a million gallons of water to produce enough lithium for a megawatt-hour of LFP batteries, while hard rock mining is highly energy intensive. Both methods can also lead to pollution by contaminating groundwater and surface water with toxic chemicals and acid mine drainage (AMD).
Finally, second-life applications reduce geopolitical risk by leveraging retired batteries that are available domestically. This is particularly relevant in the US and EU, which are highly dependent on raw material extraction and manufacturing in foreign countries.
Battery Repurposing in Practice
Second-life batteries may have tremendous economic and environmental benefits, but delivering on this potential is not straightforward. Several processes must be built to cost-effectively repurpose used batteries.
Let’s imagine that we receive a shipment of used EV battery packs. Where do we go from here?
Our first step is screening. We will turn away any batteries which do not pass our criteria and tests for mechanical, electrical, and thermal performance. Depending on where we sourced these batteries, the data may not be available to answer all of our questions, and we may need to add other screening criteria and tests to compensate.
The next step is tear-down of the battery. For the sake of this example, let’s say that we are repurposing batteries at the module level. In this case, our breakdown of the battery pack requires us to discharge the pack, drain its cooling fluid, and then mechanically break it down into individual modules.
Now we need to test our modules. Accurately testing a battery’s capacity is notoriously difficult: tests take a long time and different test stations can produce different results. This problem exists in battery manufacturing as well as repurposing, and it is attracting new solutions from second-life battery startups as well as startups that are using ultrasound and electrical impedance spectroscopy to address this challenge across the battery supply chain.
We now have a set of healthy batteries ready to be repurposed. Let’s say our end application is a 20-foot, containerized BESS. We need to consider the form factor, chemistry, and voltage of our specific battery modules to come up with the physical and electrical design of our container. The modules themselves may need some minor modifications for safe assembly. We’ll also need to design the HVAC and fire safety systems, and we will need a master battery management system (BMS) to coordinate the operation of the batteries together (more on this later).
Once the design is complete, we get to work on assembly. We build our BESS according to the design spec and run some acceptance testing to make sure it performs as needed.
Second-life BESS Performance
If you are initially skeptical of the performance of second-life batteries, you are not alone. Operating second-life batteries is a different problem than operating first-life batteries, and doing so incorrectly can quickly destroy the value of a second-life BESS. Let’s break down why performance management is so different for second-life batteries.
First, our second-life BESS will be composed of batteries with widely varying states of health. Within the same container, we may have some modules at 95% of their original capacity and some at 50%. If we don’t carefully balance these modules in real-time and over the long term, the 50% modules will inhibit the performance of the system as a whole, and the 95% modules will degrade faster than they otherwise would.
Second, some of our battery modules will eventually reach end-of-life failure modes. We will need to detect end-of-life failures and isolate those modules from the rest of our system so that they do not bring down entire strings.
Third, degradation becomes nonlinear when batteries drop below 60–70% of their original energy capacity. If our BMS uses a heuristic model for battery degradation, as most do, that will suffice before the batteries hit the nonlinear threshold, but it will exacerbate battery degradation after that threshold.
These challenges are solvable, but they require deep expertise and focused effort. At Relyion Energy, this is what we think about every day. We have built a custom BMS and associated hardware architecture to address these challenges and extend second-life battery lifetimes by decades.
The Future of Second-Life
The potential for second-life batteries is massive. At scale, second-life batteries could significantly lower BESS project costs, paving the way for broader adoption of wind and solar power and unlocking new markets and use cases for energy storage. A mature second-life ecosystem could curtail millions of metric tons of carbon emissions while materially lessening demands on natural resource production.
This post has, of course, oversimplified many things. We have barely scratched the surface of screening, testing, and design concerns involved in the safe repurposing of second-life batteries. We also haven’t touched at all on product certifications, such as UL9540, CSA 22.2, and IEC 62933, nor have we discussed the many ways that policy can help or hinder the second-life battery industry.
Many challenges remain to be solved, and an ecosystem of players is emerging to solve them: logistics companies are setting up second-life battery supply, data providers are creating systems to track the history of individual batteries, market-makers are building databases of batteries available for purchase, and system integrators are building actual second-life BESS products.
Second-life batteries will either fail or experience exponential growth over the next 3–5 years. Retired batteries are available in increasing quantities, and there is clear demand for low-cost, stationary energy storage. Companies seeking to take advantage of the opportunity must act now, or risk missing the boat.

Josh Lehman leads commercialization for Relyion Energy, a second-life energy storage company with core technology that extends lithium-ion battery life by decades. Before joining Relyion, he led product management at Stem, Inc, greatly expanding the company’s optimization capabilities for energy assets and contributing to the company’s eventual public listing. Earlier in his career, Josh built new business models that brought energy efficiency, load management, and distributed energy solutions to commercial, industrial, and low-income multifamily properties.
Relyion Energy is a member of the Volta Foundation.